Quasiparticle engineering and entanglement propagation
Quasiparticle engineering and entanglement propagation in a quantum many-body system.
P. Jurcevic, B. P. Lanyon, P. Hauke, C. Hempel, P. Zoller, R. Blatt, C. F. Roos.
Nature 511, 202 (2014)
arXiv version: PDF
The investigation of the properties of interacting quantum many-body systems is of central importance for condensed matter physics, quantum chemistry, atomic and molecular physics, and related fields. A long-standing challenge in these areas of quantum physics has been the engineering and control of quantum many-body systems with precisely tailored properties. Thanks to an exciting technological development over recent years, systems like neutral atoms, trapped ions, or photons interacting with atomic ensembles are now being used to artificially engineer such quantum matter.
A central question in this field concerns the role of genuine quantum effects, and in particular how entanglement is distributed and develops in non-equilibrium dynamics of an interacting quantum many-body system. In many cases, the quantum dynamics of a many-body system can be described by particle-like carriers of information, the so-called quasiparticles. Quasiparticles describe collective excitations of the constituents of the many-body system. Familiar examples of this concept are phonons, the lattice vibrations in a crystal, or excitons, bound states of electrons and holes in a semiconductor. The propagation of quasiparticles is governed by their dispersion relation, which determines the speed at which excitations move around the system and to what extent they stay localized.
A local perturbation of the ground state of a quantum-many body system can be described in terms of quasiparticles: an initially well-localized superposition of quasiparticles spreads out and in this process entangles the basic constituents of the quantum system.

False-colour picture of a string of 15 laser-cooled calcium ions. The ions are the basic building blocks of our quantum system. In the absence of laser light, they interact only via their mutual Coulomb repulsion. By suitably engineered laser fields, the ions can be made to interact with each other.
Engineering a quantum many-body system in a string of trapped ions
In our experiments, we artificially engineer a many-body system by trapping and laser-cooling linear strings of up to 15 ions, which are made to behave as interacting quantum magnets (see Fig. 1). Each of the ions can be in a superposition of two quantum states, which we identify with the |↑> and |↓> states of a spin-1/2 particle. In the absence of laser light, the electronic states of different ions do not interact with each other. However, by shining a suitable combination of laser fields onto the ions, an antiferromagnetic Ising-type interaction is realized: whenever one spin flips its orientation, another spin has to do likewise. By controlling the laser frequencies, we have control over the range of the interaction and can determine how fast the interaction strength decreases with increasing distance between the spins [1]. In addition, the laser fields can simulate the presence of a magnetic field that competes with the Ising interaction. In our experiment, this field is much stronger than the spin-spin coupling. As a consequence, the dynamics conserves the number of spin excitations.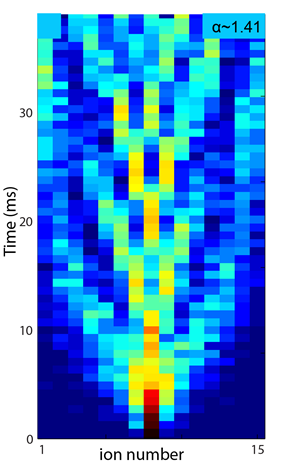
Dynamics induced by an Ising model with strong transverse field when starting from a state in which all but the center ion are in the ground state. The initially localized excitation starts to spread out to the left and right in a complicated pattern. The magnetization along the direction of the transverse field is encoded in the colour.
Experiments similar to ours investigating the dynamics following a global rather than a local quench have been recently carried out at JQI, Maryland, and published back to back with our paper [3].
Detecting entanglement in a quantum many-body system
Moreover, by measuring correlations between pairs of spins along the direction of the transverse field, we observe that propagating quasiparticles distribute correlations between the ions (if ion A is excited, ion B cannot be excited and vice versa). Similar correlations were previously observed in artificial quantum matter made of neutral atoms held in optical lattices [4]. While observing such correlations in one measurement basis is already of interest by itself, it cannot answer the question whether these correlations are of classical or quantum-mechanical origin. In our experiment, we demonstrate for the first time the quantum nature of the correlations. We achieve this by performing quantum state tomography on pairs of spins to the left and right of the initially perturbed site. These measurements reveal that the spreading quasiparticles indeed distribute entanglement among the ions.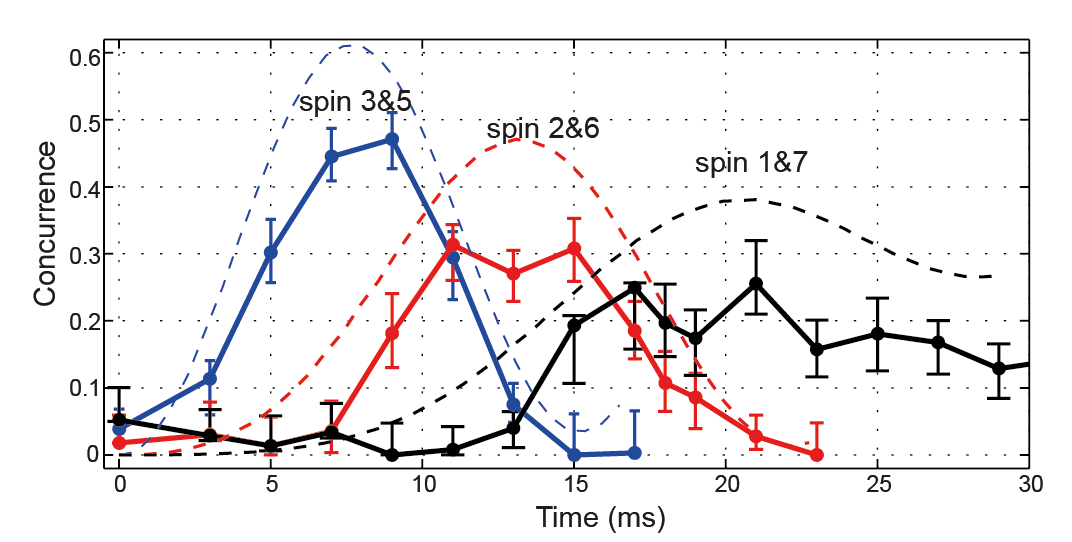
After flipping the central spin of a 7-ion crystal, quasi-particles spread out and entangle pairs to the left and right of the central spin. This entanglement is revealed by quantum state tomography of these ion pairs and shows the speed with which these quantum correlation propagate in the system.
Future experiments
What are the next steps in these experiments? While our experiment could demonstrate that pairs of ions were entangled by the Ising interaction, it remains a challenge to investigate how entanglement between larger subsystems increases the longer they interact with each other. Another important step will be to further improve the control over the quasiparticles. In most of our experiments, there was only a single quasiparticle wave-packet present. Quasiparticles, however, also interact among each other, which opens interesting perspectives for quantum-optics experiments where the fundamental objects to be studied would be quasiparticles rather than atoms, ions, or photons.
Literature
- K. Kim et al., Entanglement and tunable spin-spin couplings between trapped ions using multiple transverse modes. Phys. Rev. Lett. 103, 120502 (2009)
- P. Hauke, L. Tagliacozzo, Spread of correlations in long-range interacting quantum systems. Phys. Rev. Lett. 111, 207202 (2013)
- P. Richerme et al., Non-local propagation or correlations of correlations in long-range interacting quantum systems. Nature 511, 198 (2014)
- M. Cheneau et al., Light-cone-like spreading of correlations in a quantum many-body system. Nature 481, 484 (2012)
Financial support
This research was supported by the Austrian Academy of Science, the University of Innsbruck, the Austrian Science Fund FWF and by the European Commission via the integrated project SIQS and the project CRYTERION.
CR, July 2014